Microbe Made Fortresses Revolutionize Coastal Defenses
Biocementation—via a process known as Microbially Induced Calcite Precipitation—is a promising method for coastal defense against erosion since it is cost effective and eco-friendly compared to traditional methods such as concrete seawalls.
Reading Time: 4 minutes
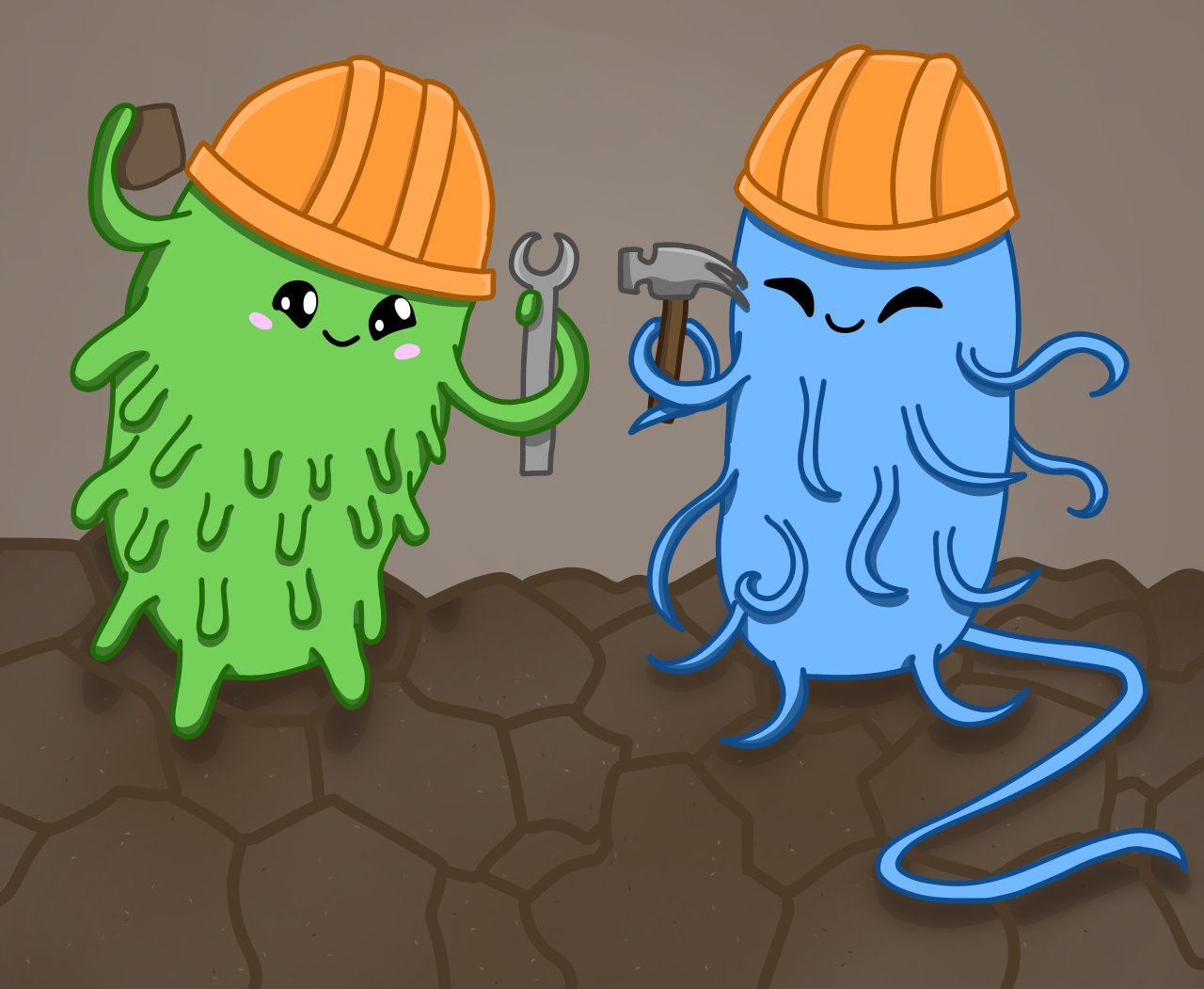
Defending our coastlines and infrastructure against erosion has been a centuries-long battle between humanity and nature. From concrete seawalls to sandbags, traditional coastal defenses have provided short-term relief. However, these defenses are environmentally and economically costly, including disruption to local ecosystems and the constant need for maintenance and replacement. But what if we could work with nature instead of fighting against it? Now, scientists are looking to the tiniest allies in the natural world—microorganisms—for a solution that’s as innovative as it is sustainable. Biocementation is a promising, cost-effective alternative to traditional coastal defense methods that will provide long-term sustainability. The solution uses microorganisms, often bacteria, to stabilize soils and combat erosion. This relies on a process called Microbially Induced Calcite Precipitation (MICP).
MICP is a biogeochemical process where microorganisms cause precipitation, or the formation of a solid from a liquid solution, of calcium carbonate in soil or other porous media. Bacteria such as Sporosarcina pasteurii produce an enzyme called urease, which catalyzes the breakdown of urea into carbonate and ammonium ions through a process called hydrolysis, where water is used to split the urea molecule. When urea is hydrolyzed, the carbonate ions bind with calcium ions, which are plentiful in soil, to precipitate calcium carbonate. Calcium carbonate is a natural “cement” that binds soil together. Another type of MICP is non-urealytic MICP. It is a rarer alternative to ureolytic MICP for soil stabilization. Unlike ureolytic MICP, non-ureolytic MICP uses a series of pathways that don’t have ammonium as a byproduct. Bacteria involved in non-ureolytic MICP include Bacillus subtilis and Bacillus amyloliquefaciens. Instead of urea, non-ureolytic MICP often uses organic salts such as calcium formate, calcium acetate, or calcium lactate as carbon and calcium sources.
Both ureolytic MICP and non-ureolytic MICP are promising coastal defense methods. They strengthen soil by binding particles together, creating a more stable structure that resists erosion—a key factor in protecting coastlines from the effects of strong waves and rising sea levels. They each have their advantages. For example, bacteria in MICP such as Sporosarcina pasteurii are naturally resilient and thrive in harsh environments such as those with high salinity or temperature changes. This means that bacteria in ureolytic MICP work better in extreme conditions. Also, ureolytic MICP generally results in more efficient calcium carbonate precipitation rates than non-ureolytic MICP. However, unlike non-ureolytic MICP, this process produces ammonium as a byproduct. Ammonium on large scales is harmful for the environment, and high levels of ammonium can lead to excessive algae growth in water bodies. This causes eutrophication, a process that depletes oxygen in the water, harming aquatic life. Thus, non-ureolytic MICP may be more eco-friendly.
Biocementation has already shown many promising applications. Researchers at the Oregon State University's O.H. Hinsdale Wave Research Laboratory conducted experiments on bio-cemented dunes. The dunes were created in a Large Wave Flume—a model of a very large and controlled “swimming pool” that replicates a piece of the ocean. Both the controlled and bio-cemented dunes went through a series of trials simulating hurricane conditions. The researchers studied the dunes’ penetration indices (PI), which measures the soil’s resistance to penetration, where lower values indicate stronger or more compacted soil. They found that the PI of the bio-cemented dunes was roughly 2.12 cm per blow, while the PI of the untreated soil was roughly 7.0 cm per blow. Another study done at the Brahmaputra river bank near the Indian Institute of Technology used biocementation to mitigate soil erosion. They stimulated the ureolytic bacteria found in the riverbank and induced MICP. The treated sand had a dramatic reduction in an erodibility test, with only 12 percent mass loss, while the untreated sand had a 56 percent mass loss. It is clear that biocementation reduces erosion and reinforces sand dunes.
Biocementation is also known to be more eco-friendly and cost-effective than traditional methods. Traditional methods such as concrete barriers and seawalls can be costly, environmentally disruptive, and require significant maintenance. A life cycle assessment found that biocementation has 90 percent less abiotic depletion potential—a measure of the depletion of non-renewable natural resources due to human extraction and consumption—and three percent less global warming potential compared to Portland cement used in soil stabilization. Natural defenses such as biocementation can be two to five times cheaper than submerged breakwater defenses, which are offshore structures that reduce wave heights. Biocementation has also been proven to be adaptable, working in various types of soil such as sand, sandy soil, sloped soil, and poorly graded soil.
Although biocementation has great promise, implementation is still challenging. One issue is uneven distribution of cementation. A study found that clogging at injection sites in the top 10 centimeters of soil causes the calcium carbonate to form and distribute unevenly. Researchers found that the site of injection had a 35 to 75 percent concentration of calcium carbonate, while the middle and lower regions only had about 10 to 26 percent—meaning there was heavier precipitation at the top. Heavier precipitation of calcium carbonate at the top can create a hard surface layer that prevents proper stabilization of the deeper soil, weakening the overall effectiveness of biocementation. Another issue is the microbe used. The performance and efficiency of biocementation often varies by pH, temperature, and nutrients. Researchers are still working to optimize these bacteria in harsh conditions.
Overall, biocementation may be the future of our coastal defense. In comparison to traditional methods, it is cheaper and more eco-friendly in the long term, while being just as efficient. Given Stuyvesant’s proximity to the Hudson River and the growing threat of rising sea levels in New York City, it is increasingly critical to explore innovative and sustainable defense strategies like biocementation.